Ion
lasers deliver power at visible and UV wavelengths
Jeff Hecht,
Contributing Editor
Laser Focus World |
The ability to generate continuous-wave (CW) powers of several milliwatts up to tens of watts at a variety of visible wavelengths, and up to several watts in the ultraviolet (UV), has made rare-gas ion lasers useful in applications from pumping tunable lasers to putting on laser light shows. They are used regularly to detect latent fingerprints, create masters of video disks and compact disks, make medical measurements, and perform fluorescence studies. New applications for ion lasers continue to emerge, including producing three-dimensional (3-D) models of parts in a process called stereolithography and serving as light sources in confocal microscopes.
Engineers have steadily enhanced the performance of rare-gas ion lasers. Lifetime, durability, and output power have improved, while costs have been reduced and shorter UV wavelengths made available. Limited efficiency of ion lasers remains a serious drawback, and developers of diodepumped neodymium lasers have set their sights on replacing low-power argon lasers with frequency-doubled Nd:YAG lasers. But they are not there yet. The high cost of pump diodes leaves low-power ion lasers with healthy price advantage, and frequency-doubled Nd:YAG lasers have yet to match the highest powers from ion lasers. Meanwhile, other solid-state laser advances have helped create a new market for ion lasers-pumping tunable Ti:sapphire lasers.
Many lasers operate on ionic transitions, but an ion laser is normally meant to be one in which the active medium is a an ionized rare gas. The most important com mercial ion laser is based on ar gon, with strong emission lines in the blue-green and weaker lines in the UV and near-IR (see table). Krypton lasers have weaker output but produce a broader range of visible wavelengths, plus additional LJV lines. Mixing argon and krypton gas produces a multigas laser that emits on lines of both gases.
Ion lasers are excited by passing a high-current discharge through a tube containing about 1 torr of pure laser ps or a mixture of argon and krypton in mixed-gas lasers. The discharge is concentrated in a small-diameter bore at the center of the tube, which is where laser action occurs. An initial spike of a few thousand volts breaks down the gas, then voltage drops to 90-400 V while the current jumps to 10-70 A in the sustained discharge. An external magnet producing a magnetic field parallel to the bore axis can help confine the discharge to the bore.
|
Single-line
output powers on major argon and krypton lines
|
Wavelength
(nm) |
Relative
power (compared to multiline argon visible output) |
Line
power
(W)
|
Argon |
275.4 |
0.064
(combined) |
1.6 |
300.3 |
302.4 |
305.5 |
334.0 |
0.28
(combined) |
7.0 |
351.1 |
351.4 |
363.8 |
454.6 |
0.03 |
0.8 |
457.9 |
0.06 |
1.5 |
465.8 |
0.03 |
0.8 |
472.7 |
0.05 |
1.3 |
476.5 |
0.12 |
3.0 |
488.0 |
0.32 |
8.0 |
496.5 |
0.12 |
3.0 |
503.7 |
0.07 |
1.8 |
514.5 |
0.40 |
10.0 |
528.7 |
0.07 |
1.8 |
Krypton |
337.5 |
0.08
(combined) |
2.0 |
350.7 |
356.4 |
406.7 |
0.036 |
0.9 |
413.1 |
0.07 |
1.8 |
415.4 |
0.01 |
0.28 |
468.0 |
0.02 |
0.5 |
476.2 |
0.016 |
0.4 |
482.5 |
0.016 |
0.4 |
520.8 |
0.028 |
0.7 |
530.9 |
0.06 |
1.5 |
568.2 |
0.044 |
1.1 |
647.1 |
0.14 |
3.5 |
676.4 |
0.036 |
0.9 |
752.5 |
0.048 |
1.2 |
793-799.3 |
0.12 |
0.3 |
|
Laser action takes place on transitions far above the ground level of the ion and even further above the neutral ground state (see Fig. 1). The two principal visible argon lines are 514.5 nm (green), which is stronger in larger lasers, and 488 nm (blue), which is stronger in smaller air-cooled argon lasers. Argon lasers are used in a variety of applications requiring visible light, including pumping of tunable lasers, reprographics, displays, and mastering of videodisks. Singly ionized argon
(Ar , or Ar II in spectroscopic notation) also produces several weaker blue-green laser lines and a near-infrared line. Argon ions in the lower laser level release energy by spontaneous emission in the far ultraviolet and drop to the ion ground state. This energy-level structure limits the wall-plug efficiency of visible argon lasers to no more than 0.1%.
Higher discharge currents can produce doubly ionized argon
(Ar , or Ar
III), which has UV laser lines. The most useful UV lines are at 275, 300-306, 334, 351, and 364 nm. Because higher discharge currents are needed, the wall-plug efficiency of these lasers is limited to 0.01%. The shorter lifetime of the UV argon-ion laser is also a result of the high discharge current. Harmonic generation of the visible argon lines can yield even shorter wavelengths.
Krypton generates only 10%-30% as much power as argon used in the same tube. The strongest line of singly ionized krypton
(Kr ) is at 647.1 nm (red), but yellow, green, and violet lines can produce up to one half as much power, as shown in the table. The violet lines are important for dyelaser pumping; the red and yellow lines are important for displays. Doubly ionized krypton
(Kr ) has three near-UV lines. Laboratory lasers apparently operating on
Kr lines have generated CW powers totaling 2.5 W at 242 to 266 nm and wavelengths as short as 219
nm.1 These lasers, however, have yet to be commercialized.
Mixed-gas lasers are mainly used in displays and entertainment, where it is important to balance output of the red, yellow, and blue-green primary lines. Early mixed-gas lasers lost krypton faster than argon. The yellow line would fade first, then the red line, so after 1000 h, what started as a mixedgas laser behaved more like an argon laser. Recent improvements, including sealing hard-coated mirrors directly to the tube and adding gas-fill systems, have extended multigas laser lifetime to thousands of hours.
|
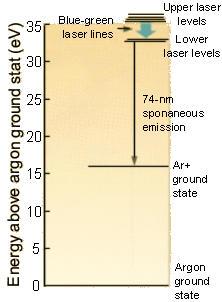 |
FIGURE 1.
Energy levels of singly ionized argon (Ar'), simplified to show only
the major bluegreen transition, are far above the neutral ground
state. Lower laser level drops to the ground state of Ar'
spontaneously with emission of a 74-nm extreme ultraviolet photon. |
FIGURE 2.
(seen below)
Typical low-power air-cooled argon-ion-laser tube shows characteristic long discharge bore and fat cathode region, which serves as a gas reservoir. Air removes excess heat from cooling fins attached to the tube.
|
Another rare gas
that can be operated as an ion laser is neon. Singly ionized neon (Ne )
can emit 1 W of CW multiline UV, divided among three lines: 332.4 nm (0.5
W), 337.8 nm (0.2 W), and 339.2 nm (0.3 W). The HeNe laser operates on
neutral neon lines, so it is not an ion laser. The only ion laser that
normally operates pulsed is xenon, which emits at several lines between
488 and 540 nm. Neon- and xenon-ion lasers are available commercially, but
are not in wide use.
Plasma-tube design
The conditions inside an ion-laser plasma tube are extremely harsh. High current densities lead to sputtering of materials in the laser tube and bore, eroding the surfaces, contaminating gases, and trapping some gas atoms. The strong electron flow in the discharge pushes neutral atoms toward the positively charged anode, while positively charged ions migrate toward the negative cathode. The inefficiency of laser operation generates waste heat that must be removed from the laser tube. Much of the relaxation energy appears as short-wavelength UV radiation, which can damage optical surfaces and glasses. Laser engineers have used various approaches to solving these problems.
To achieve reasonable lifetimes, ionlaser tubes are made of ceramic or metal-ceramic structures. For low- to moderate-power operation, a typical design has a beryllia (BeO) bore, with Brewster-angle windows that couple to external optics (see Fig. 2). Reserve gas is kept in a reservoir, which can be an expanded region at the end of the bore or a separate tank. Excess heat is removed by blowing air over the tube or flowing water along its ceramic outer jacket.
|
For
higher-power ion-laser operation, metal disks are brazed inside a
large-diameter ceramic (usually alumina, A1 O ) tube (see figure
at top of page). Central holes in the metal disk define the laser bore,
concentrating current flow where laser action occurs. In this
design, tungsten metal disks are bonded to copper cups that
transfer heat to the ceramic tube. Holes in the disks allow gas
flow within the laser tube. Water flowing along the outside of the
ceramic tube removes excess heat.
Cooling is critical to ion-laser operation. Forced-air cooling is possible for lasers operating at low to moderate powers-usually up to a few watts multiline from argon lasers. A fan blows air across the laser tube, which is normally equipped with cooling fins. Air cooling avoids the need for plumbing, thus simplifying installation and use, but it can induce vibrations that may degrade beam quality and stability. Closed-cycle water cooling is an alternative for operation at moderate powers; water flowing across the laser tube transfers energy to a heat exchanger that dumps it to the air. Only open-cycle water cooling can remove the heat generated by higher-power lasers. This configuration requires plumbing but avoids any vibration problems.
Ion-laser
tubes range from less than 20 cm long to about 2 m long.
Air-cooled lasers that generate powers of several milliwatts have
the smallest tubes, typically about 30-50 cm long. Higher-power
water cooled lasers typically come in two sizes,
"small-frame" lasers with 1-m tubes and
"large-frame" lasers with 2-m tubes.
Optics
Many ion-laser tubes have Brewsterangle windows, one fused to each end which transmit linearly polarized light to external optics. Intense shortwavelength UV light from the laser plasma can form defects in silica window materials. Although fused silica can be used in low-power visible lasers, crystalline quartz is needed for high powers or UV lasers because it suffers less damage. Protective coatings inside the Brewster window also reduce damage.
Cavity optics select oscillation wavelengths from among the many ion-laser lines. Standard optics are reflective over much of the visible spectrum, generating multiline oscillation at blue-green wavelengths from argon lasers or at most or all of the visible wavelengths with krypton. Different optics are needed for UV operation.
External optics allow users to adjust the laser so it can operate on different lines or on single lines, but they add to the complexity. External optics options include tuning prisms to select single lines, etalons for single-frequency operation, and servo loops for frequency stabilization. Alternatively, lasers are available with cavity mirrors integral with the tube, simplifying operation but limiting output to preset lines (often multiline emission). Sealing hard-coated optics directly to the tube, rather than using Brewster windows, can extend tube lifetime, although at the cost of limiting operating options.
Laser operation and lifetime
Argon, krypton, and mixed-gas lasers generate CW beams ranging from a few milliwatts to tens of watts. Highest powers are produced by watercooled argon lasers in multiline visible operation. Ion lasers can be modelocked to produce trains of picosecond pulses, which typically are used to pump tunable dye or solid-state lasers. Cavity dumping can slow the repetition rate and increase the peak power of modelocked pulses.
Frequency doubling of the visible argon-laser lines can produce CW powers of hundreds of milliwatts at 229-257 run, wavelengths that otherwise are difficult to generate. Intracavity doubling takes advantage of the high power circulating within the cavity to generate the highest powers. To date, B-barium borate
(BBO) in an argon laser customized for second-harmonic operation has produced frequency-doubled powers of 750 mW at 257 nm and lower values at other wavelengths.
Early ion-laser tubes were fragile and short-lived. Tube lifetime and durability have improved, although some care is still needed in handling. Specified lifetimes range from about 2000 to 10,000 h, but actual operating times can reach tens of thousands of hours. One industrial user reports that average tube lifetimes increased from about 1500 h in tubes installed before mid-1986 to 5400 h today.
(continued top of next column)
|
Applications
The blue-green output of argon lasers is valuable because many materials respond to it much more strongly than they do to the less-energetic red photons from other lasers. Argon lasers are used in printing and publishing applications, including high-speed computer output printing, exposure of printing plates and films, and production of halftone patterns during color separation. (Ultraviolet ion lasers also are used to expose some printing plates and films.) Argon lasers write data on the master disks used for replication of video disks, audio compact disks, and CD-ROMs for computer data retrieval. An emerging application is writing laser alignment marks on special 3.5-in. magnetic disks in what is called
"floptical" format; the laser marks control position of read/write heads so 20 Mbit of data can be stored on the disk.
Visible argon lasers also fit the requirements of many measurement applications. They can be used in laser Doppler
velocimetry, laser Doppler anemometry, and particle sizing. Visible argon lines stimulate fluorescence from oils found in human fingerprints, so these lasers can be used in forensic analysis.
Both visible and UV argon-ion lines are used to stimulate other molecules to fluoresce in general research and for biomedical use in studying cell structure and DNA. For example, cell researchers bind fluorescent dyes to structures within the cell, illuminate them with visible argon lines, and observe the fluorescing structures against the dark background of the rest of the cell. By binding the fluorescent dyes to antibodies, they can highlight specific proteins that react with the antibodies.
Ultraviolet ion lasers can be used in stereolithography systems, in which 3-D models are built up from computer designs layer by layer. Laser light is focused onto a platform in a bath of liquid polymer that solidifies on exposure to UV light. The platform is placed just below the surface as the laser traces the pattern of one layer of the part. Then the platform is lowered for the laser to trace the next layer. Layer by layer, the system builds a 3D model that engineers can use in prototype development.
Ophthalmologists have long used argon lasers for photocoagulation of the defective retinal blood vessels that impair vision in diabetics. Recently, a portable 65-lb system has been developed that fits into a wheeled suitcase and requires only 115-V power. Ion lasers are also used for illumination in confocal microscopes, which give better optical resolution than conventional microscopes.
Tunable Ti:sapphire lasers have created a new niche for argon lasers because the visible lines fall in the Ti:sapphire pump band. Argon lasers are also a standard pump source for tunable dye lasers. The violet lines of krypton and the UV argon lines are used to pump shorter-wavelength dyes. Because dye degradation becomes a bigger problem at shorter wavelengths, researchers prefer to use the longest wavelengths suitable for pumping dyes.
Improvements in mixed-gas laser lifetimes have led to increasing interest in their use for displays and light shows. The combination of the red and yellow krypton lines and the bluegreen argon lines gives reasonable color balance for large-area displays. Enhanced reliability has increased sales for entertainment, where few operators are trained to deal with laser problems.
Ion lasers also are used in holographic nondestructive testing. For example, with I W of CW singlefrequency output, ion lasers can record a hologram of an entire 6- or 8-in. semiconductor wafer. This process lets chip makers check the flatness of a wafer before fabricating integrated circuits on it.
The range of ion-laser applications continues to broaden. While ion lasers may not be ideal tools, they are versatile and valuable, providing the highest CW powers on the market at visible and near-UV wavelengths. They operate in a variety of modes, from generating high levels of raw power for laser pumping and fluorescence stimulation to producing single-frequency beams for velocimetry and Brillouin spectroscopy.
ACKNOWLEDGMENT
This material was adapted with permission from The Laser
Guidebook, 2nd ed., J. Hecht (McGraw-Hill, New York, NY,
1992). Ordering information can be obtained by calling
1-800-2MCGRAW.
REFERENCE
1. A. B. Peterson, Proc. CLEO '92, Technical Digest 7,
paper CTUH10, Anaheim, CA (May 1990).
|
This
article was reproduced by Laser Innovations with permission.
2001 |
Press
the back button on your browser to return to the previous page. |
|
|